Erythrocytes, also known as red blood cells, are the most abundant and the smallest (at least in mammals) blood cells. Their main function is transporting O2 and CO2 between the lungs and the rest of the body. In humans, the number of erythrocytes is lower in females, around 4.6 million/mm3, than in males, about 5 million/mm3. These numbers are higher in humans living in places with lower amount of oxygen, such as at high altitude regions. In fresh blood, erythrocytes show a red-orange color, that is why the name erythrocyte. The color is a consequence of the high content of hemoglobin, the protein that is also responsible for the red color of the blood. Erythrocytes rarely leave the blood stream.
1. Morphology
In vertebrates, the shape of erythrocytes is variable. They are biconcave in mammals, with a thinner central region because they have no nucleus (Figures 1 and 2). They are 8 µm in diameter and 2 µm thick in the thicker part. Erythrocytes have no organelles, and they lack transcellular cytoskeleton (that is, in those regions not close to the plasma membrane). They cannot synthesize new proteins, but contain about 450 mg/ml of hemoglobin. Hemoglobin is a globular protein consisting of 4 polypeptide chains, each having a heme group and an iron atom in the center of the heme group. The iron is able to transport O2 and CO2. The biconcave morphology of erythrocytes provides a high surface/volume ratio, increasing the efficiency of the O2 and CO2 diffusion across the plasma membrane. In non-mammal vertebrates, erythrocytes have an ellipsoid and biconvex shape because they have a nucleus with condensed chromatin (Figure 3).
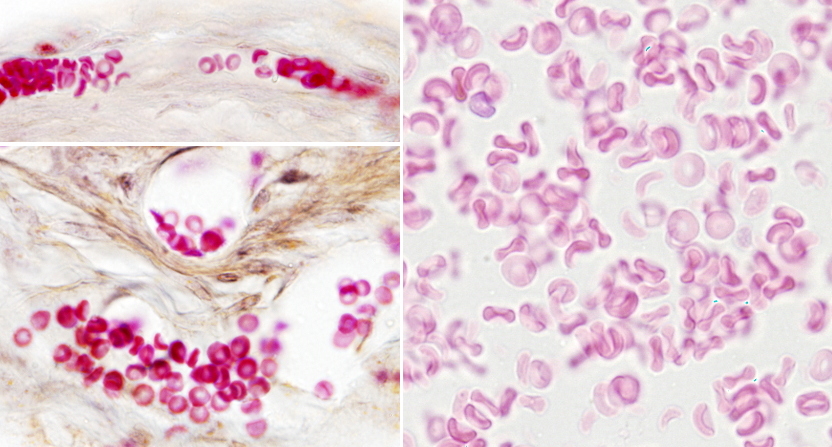

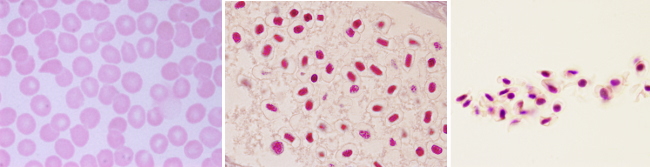
Erythrocytes from different animal species show a variety ofdiameters. It is about 50 µm in some amphibians, whereas in mammals is about 10 µm. In mammals, the diameter of capillaries is sometimes smaller than 10 µm so that erythrocytes have to fold to go through the narrowest vessels. This would be very difficult for a nucleated erythrocyte, and it is thought that the lost of the nucleus is an evolutionary advantage that increases the flux stream of blood through smaller blood vessels and decreases the probability of having thrombosis (plugged up). One may think that lacking a nucleus leaves more space for hemoglobin, but there are other strategies to increase the intracellular amount of hemoglobin. For example, in birds, there is hemoglobin inside the nucleus.
The biconcave shape of mammal erythrocytes is stable in normal conditions, but it may also be remarkably twisted when erythrocytes go through small capillaries. Capillaries may be 3 µm in diameter, a size smaller than an erythrocyte. Furthermore, erythrocytes have to withstand strong mechanical forces from blood stream in large arteries. Nonetheless, erythrocytes are really stable cells because of their cellular features. The plasma membrane of erythrocytes shows a cholesterol proportion above 30 %, higher than in an average cell, which makes plasma membrane less fluid, more stiff and more hydrophobe (less permeable). Therefore, the plasma membrane is more resistant and well-sealed, making breakages more difficult and preventing the lost of inner content. Furthermore, the plasma membrane is anchored to a cytoskeleton scaffold of about 100 nm thick that covers its inner surface (cytosolic). This network makes possible to resist and respond to changes in cell morphology.

The cytoskeleton beneath the plasma membrane is made up of transmembrane glycoproteins, such as glycophorins (there are five types), and band proteins 3, 4 and 5, as well as an associated network of spectrin, ankyrin and actin (Figure 4). Spectrin consists of two subunits, alpha and beta, that intertwine between each other to form alpha-helix filaments. These filaments are anchored to the plasma membrane by attachments to glycophorins and band 3 transmembrane proteins.
The extracellular domains of glycophorin A are glycosylated and determine the blood type. There are more than 35 blood types, most of them are very rare. For blood transfusions, only ABO and Rh antigens are considered. The ABO group consists of two antigens: A and B, whereas O blood type lacks both. Rh antigen can be present or not, so there are Rh+ and Rh-, respectively. Band transmembrane proteins are transporters. Ban 3 is an exchange transporter of carbonate/chloride, and Band 4 and 5 are glucose transporters.
2. Origin and distribution
In adult mammals, erythrocytes are generated by a process known as erythropoiesis. It takes place in the bone marrow from precursor cells. In the fetus, the production of erythrocytes happens in the spleen due to the lack of bone, and therefore of bone marrow. In adults, under some circumstances, erythropoiesis may also happen outside the bone marrow. For example, it has been found to take place in the liver, spleen, lymphatic ganglia, and paravertebral region.
The erythropoiesis takes place in cellular clusters known as erythorblastic islands, consisting of erythroblasts surrounded by a macrophage (Figure 5). The macrophage is essential for the differentiation process. In addition, it synthesizes ferritin, which is necessary for hemoglobin synthesis. The erythropoiesis can be divided in two phases: the proliferation of progenitor cells and the differentiation pathway, which begins with the proerythroblasts, the first cell that cannot longer proliferate.

The erythropoiesis begins when a myeloid adult stem cell differentiates into the BUF-E (burst-forming unit-erythroid) progenitor cell (Figure 5). BFU-E is the first of the cell lineage committed to produce erythrocytes (erythrocyte lineage). These cells proliferate and differentiate into the CFU-E (colony-forming unit-erythroid) progenitor cell. BUF-E is the most proliferating cell type, followed by CFU-E cells. During this early period, progenitor cells are dependent on erythropoietin. CFU-E cells first differentiate into proerythroblasts, proerythroblasts into erythroblasts, and erythroblasts into mature erythrocytes. During the differentiation process, there are changes in shape and cell size (Figure 5), hemoglobin content and composition, plasma membrane structure and function, nuclear variations, and other cell transformations. In mammals, the nucleus, Golgi apparatus, mitochondria, and centrioles are lost. Chromatin is condensed, the nucleus reduces its volume to 1/10 of the initial size. The nucleus and perinuclear cytoplasm are finally eliminated during an asymmetrical division and then phagocyted by a macrophage. Although the nucleus becomes condensed in all vertebrate species, it is expelled only in mammals. During differentiation, hemoglobin related genes are over-expressed, as well as those involved in plasma membrane and cytoskeleton behavior.
Erythropoietin is the main cytokine involved in the production of erythrocytes. In mammals, it is released by the kidneys after induction by low levels of oxygen in the blood. Eyrhtropoietin is recognized by CFU-E cell receptors and has two-fold functions: preventing apoptosis and inducing proliferation and maturation into proerythroblasts. Each CFU-E cell gives rise to about 30 to 40 mature erythrocytes.
Erythrocytes enter the blood stream as differentiated cells, and their average life is 120-140 days in humans (more than 5 million erythrocytes die every second). In turtles, the average life of erythrocytes is 10-11 months, whereas in mice is 40 days. Erythrocytes die as they consume their set of enzymes and are removed from the blood stream by macrophages, mainly by Kupffer cells in the liver and by machrophages in the spleen. These two organs are capable of recycling several waste products that result from hemoglobin degradation. The detection and phagocytosis of aged erythrocytes may depend on the sialic acid lost from the erythrocyte glycocalyx and on some other carbohydrates and markers exposed in the cell surface, which are recognized by immunoglobulins, and then by macrophages. Another feature of aged erythrocytes is that they alter the plasma membrane asymmetry so that some lipids of the inner monolayer are exposed at the outer monolayer. During erythrocyte aging, there is a reduction of cell size and an increase of the cytoplasm density. It may be a consequence of the cytoskeleton disorganization, leading to a fragmentation of the cell in small vesicles.
3. Functions
The biconcave shape of mammalian erythrocytes provides a large surface/volume rate that improves their main function: transport and exchange of O2 and CO2 in the lungs and in the rest of the body. Hemoglobin combines with O2 in the lungs to form oxyhemoglobin. As erythrocytes go through the body organs, they release the O2 by concentration gradient. The total oxyhemoglobin of an erythrocyte may transport millions of O2 molecules. There are 4 heme groups in every hemoglobin molecule, and each heme group transports one O2 molecule. About 280 million of hemoglobin proteins are estimated to be within each erythrocyte. Deoxyhemoglobin is the name of hemoglobin without O2. It gives a darker red color to the blood.
Hemoglobin also transports CO2, which diffuses from the tissues to the bloodstream. Hemoglobin loaded with CO2 is known as carbaminohemoglobin, which travels to the lungs to release CO2 is released. Gases diffusion is by concentration gradient. In body tissues with low O2 concentration and high CO2 concentration, O2 is released from hemoglobin and CO2 is loaded. O2 is fetched and CO2 is released in the lungs.
Blood clotting, hemostasis and thrombosis have not been traditional related to platelets and blood plasma, but not to erythrocytes. However, many evidences point to erythrocytes in these processes. A low hematocrit (low erythrocyte density) is related to a longer bleeding time, which is independent on platelet density. High hematocrit is more prone to thrombosis. Erythrocytes contribute to blood viscosity that increases the probability of thrombosis. When hematocrit rises, erythrocytes tend to occupy the center of the blood vessel, pushing platelets and plasma to the periphery, close to the endothelium. This disposition is good for larger blood vessels, but it is risky in small capillaries because the blood viscosity increases. In addition, nitric oxide is released in less amount by the endothelium so that vasodilation is not activated. Erythrocyte stiffness caused by some pathologies (autoimmune hemolytic anemia, thalassemia, xerocytosis, etcetera) leads to a higher risk of thrombosis because erythrocytes go through small capillaries with more difficulty.
The presence of phosphatidylserine in the surface of platelets is important for blood clotting. In the outer surface, phosphatidylserine can be associated with clotting molecules. The movement of phosphatidylserine from the inner monolayer to the outer monolayer of the plasma membrane is driven by proteins like flippases and scramblases when calcium enters platelets. The same process also happens in erythrocytes. In erythrocytes, the transport of phosphatidylserine to the outer monolayer is the starting point for apoptosis. However, only 0.5 to 0.6 % of erythrocytes start apoptosis at the same time, so that the thrombotic activity of this process is low. During some pathologies, the proportion of apoptotic erythrocytes may be higher, therefore increasing their influence in thrombosis. In addition, as other cells, erythrocytes are also able to release extracellular vesicles during apoptosis, aging and pathological processes. These vesicles are released when the membrane asymmetry changes (exchange of lipids between both monolayers) and increases the thrombosis probability. When blood is stored for a long time, erythrocytes get more rigid, start apoptosis more easily and release many extracellular vesicles.
Erythrocytes influence blood clotting and thrombosis in other ways. Hemolisis, or erythrocyte breakage, is also harmful because it favors thrombosis by releasing microvesicles and hemoglobin into the bloodstream. Nitric oxide is a vasodilator, and hemoglobin removes nitric oxide from blood plasma. During normal conditions, there is little interaction between erythrocytes and endothelium. However, in some pathologies, the adhesion of erythrocytes to endothelial cells may be higher, increasing the probability of blood vessel obstruction. Furthermore, during blood clotting, fibrinogen and platelets may gather erythrocytes so that erythrocytes increase the consistence and decrease the permeability of the blood clot.
4. Pathology
The most common pathology associated to erythrocytes is anemia, which includes a wide variety of diseases. The elements for detection of anemia are the amount of hemoglobin (hemoglobin per erythrocyte) and mean corpuscular volume (MCV; mean erythrocyte volume). MCV is calculated as follows:
MCV=(Hct/RBC)*10. Hct: hematocrit (volume of erythrocytes out of the total blood volume) y RBC: number of erythrocytes in one milliliter (Figure 6).
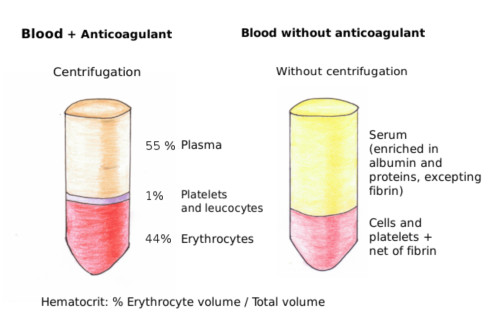
The normal hematocrit is actually a range of values. The normal values depend on the age, sex, and the altitude where the people are living. Any value lower or higher than the values of the normal interval means a decrease in the O2 and CO2 transport in the organism. There are several types of anemia:
Ferropenic anemia: lack of iron. It is caused by shortage of iron intake, either by low absorption in the intestine or by blood lost (hemorrhage). Blood smears from ferropenic anemia show too hypochromic (low pigmented) and microcytic (low size) erythrocytes. Erythrocytes are also variable in size.
Megaloblastic anemia: folic acid or B12 vitamin deficiency. Hypovitaminosis affects erythrocyte precursor cells by reducing their capability for synthesizing DNA, therefore inhibiting replication. The cell cycle stops before mitosis and the cell keeps growing, but it is not able to divide. That is why it is also known as macrocytic anemia. It is characterized by large, non-mature, and non-functional cells with nucleus, that results in a reduced erythrocyte production. Hypersegmented neutrophils are a feature of this disease.
Hemolytic anemia. It is divided in two types. Acquired hemolytic anemia, that can be immune, and hereditary hemolytic anemia, which is the most frequent. Malformation and partial or total breakage of erythrocytes occur in hereditary hemolytic anemia. This disease may be caused by failures of cytoskeleton proteins, erythrocyte enzymes and hemoglobin itself (thalassemia). Hereditary spherocytosis is characterized by a decrease in the surface/volume ratio of the erythrocytes, which leads to cell fragility and hemolysis. In blood smears, erythrocytes lack the typical faint central zone.
-
Bibliography ↷
-
Bratosin D, Mazurier J, Tissier JP, Estaquier J, Huart JJ, Ameisen JC, Aminoff D, Montreuil J. 1998. Cellular and molecular mechanisms of senescent erythrocyte phagocytosis by macrophagues. Biochemie. 80: 173-195.
Carr JH, Rodak BF. 2010. Atlas de Hematología clínica. Ed. Panamericana. 3ed. Argentina.
Doohan J. SBCC (consultada en noviembre 2014) http://www.biosbcc.net/doohan/sample/htm/Blood%20cells.htm
Ji P, Murata-Hori M, Lodish HF. 2011. Formation of mammalian erythrocytes: chromatin condensation and enucleation. Trends in cell biology. 21: 409-415.
Lux IV SE. 2016. Anatomy of the red cell membrane skeleton: unanswered questions. Blood. 127:2
Ruíz Argüelles GJ. 2009. Fundamentos hematología. Ed Panamericana 4ed. Argentina.
Nigra AD, Casale CH, Santander VS. 2020. Human erythrocytes: cytoskeleton and its origin. Cellular and molecular life sciences. 77: 1681-1694.
Ruíz Argüelles GJ. 2009. Fundamentos hematología. Ed Panamericana 4ed. Argentina.
Weisel J W, Litvinov RI. 2018. Red blood cells: the forgotten player in hemostasis and thrombosis. Journal of thrombosis and haemostasis. 17: 1–12.
-