1. Morphology
2. Source and proliferation
- Vasculogenesis
- Angiogenesis
3. Function
- Barrier
- Blood properties
- Defense / immune
At the beginning of the XIX century, von Reckingausen observed that blood vessels were lined by a layer of cells. This one-cell thick layer is made up of endothelial cells that constitute the surface of blood and lymphatic vessels. In humans, it is estimated that the total endothelial layer is about 350 m2 and that there are around 1 to 1,6*1013 endothelial cells. The total length of arteries, veins, and capillaries is about 90000 km (more than traveling twice around the world). Endothelial cells are flat and attached to one another by cell junctions. Their main function is as intermediaries between the blood, or lymph, and the other tissues, and contribute to blood properties, both in normal and pathology tissues. They are also important for the exchange of immune cells between the blood and tissues.
1. Morphology
Endothelial cells are very flattened cells, so much that their nucleus is the taller structure of the cell, even if the nucleus is flattened too. The cellular shape follows the duct morphology (Figures 1, 2, and 3). In the more narrow capillaries, an endothelial cell may extend the cytoplasm along all the perimeter of the blood vessel, so that the duct is a row of endothelial cells. In larger ducts, like arteries and veins, many endothelial cells are needed for covering the total perimeter of the duct.

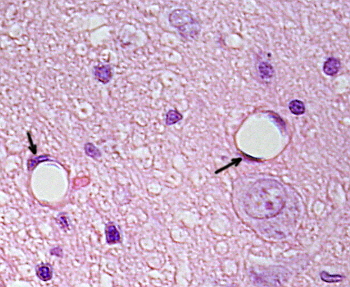
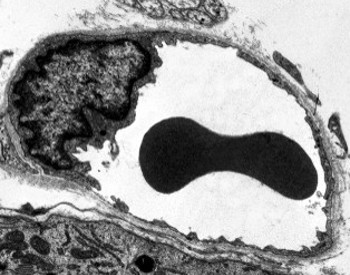
Perinuclear cytoplasm of endothelial cells contains most of the mitochondria and other organelles, such as the Golgi apparatus. The peripheral cytoplasm is scarce and has few organelles, although endoplasmic reticulum tubules can be found close to the plasma membrane. Glucose is the main energy source of endothelial cells, but metabolized by the glucolitic pathway. Hence, the low mitochondrial number in these cells. However, there are some endothelial cells with more mitochondria, such as the central nervous system endothelium, because they need more energy for a more intense transport. One feature of endothelial cells is that they have many vesicles, most of them are endocytic vesicles.
In some regions, such as liver, kidney cortex, and endocrine glands, the endothelial cells in capillaries show pores or small passages, known as fenestratrions. They are pathways of about 60 to 70 nm in diameter, with a narrow passage of about 5 nm, that directly communicate the blood, or lymph, with surrounding tissues. Capillaries having these type of endothelial cells are known as fenestrated capillaries (see below: Figure 4). Pores are distributed in groups and the density depends on the type of endothelium. Continuous capillaries are those that do not show these pores.
Endothelial cells are separated from the surrounding tissue by a layer of extracellular matrix called basal lamina. They contribute to this layer synthesizing proteins, such as laminin, fibronectin, and type II, IV and V collagens. The apical surface of the endotheliall cells is covered by a layer of carbohydrates known as glycocalyx. It forms a negative electrically charged layer that works as a barrier between the liquid and the membrane of the endothelial cells. Proteoglycans, glycosaminoglycans, glycoproteins and glycolipids form the glycocalyx. Glycosaminoglycans are the more abundant carbohydrates in the endothelial glycocalyx. Heparan sulfate and hyaluronic acid account for more than 90 % of the glycocalyx carbohydrates. Some proteins (albumin, orosomucoid, anti-trombin III, superoxide dismutase, lipases, growth factors, chemochines, and others) of the blood plasma are associated with the glycocalyx forming an additional layer. Glycocalyx is renewed and adapted to the physiological state of the cell. The hyaluronic acid is released by the endothelial cells, but it remains attached to the cell membrane, or is removed from the plasma by kidneys and liver. The hyaluronic acid is recognized by the CD44 receptors found in the plasma membrane, and organized into hexagonal structures on the free surface of endothelial cells. However, the heparan sulfate is arranged perpendicularly to the cell surface.
2. Source and proliferation
Vasculogenesis is the formation of new endothelial cells in tissues with no preexisting blood vessels. It happens in the embryo, where the blood system is one of the earlier system to be formed. In adults, and also in embryos, new blood vessels, and therefore new endothelial cells, are generated from already present blood vessels. This process is known as angiogenesis. New endothelial cells are produced by branching or dividing preexisting blood vessels. Angiogenesis is common in growing tissues and in those under heavy remodeling, such as the uterus in mammal females, and in pathologies, such as tumors, inflammation and wounds.
Vasculogenesis
In mammals, the earliest endothelial cells are generated after gastrulation during the embryo development. They differentiate from cells called angioblasts, concurrently with their arrangement into short blood vessels. This process first happens in the vitellin sac of the embryo. These initial blood vessels grow and connect between one another to form a network. Later, the these blood vessels recruit fibroblasts and muscle cells. The initial network undergoes a continuous remodeling process during these developmental stages. In embryos, all endothelial cells are similar, but they get progressively differentiated in endothelium of veins, arteries, capillaries and lymphatic vessels. Several molecules, such as FGF2, BMP4, IHH and VEGF, induce separated differentiation pathways. Notch inducer appears to be very important for the development of the artery endothelium. But, when it is inhibited, it leads to vein endothelium. Lymphatic endothelium is formed from the cardinal vein endothelium and needs the expression of COUPF-II and SOX18. In addition, the endothelium is differentiated accordingly to the organ. For example, the endothelium forms a tightly sealed layer in the brain as part of the blood-brain barrier, but it is fenestrated or loosely organized in the liver to favor the exchange of molecules across the capillary wall.
As endothelial cells are getting organized in artery and vein ducts, some endothelial cells give rise to hematopoietic cells by a differentiation process known as endothelial-hematopoietic transition (EHT). This process also happens in some adult tissues. For example, during the formation of placenta and in other tissues during pregnancy.
Angiogenesis
AAngiogenesis is the formation of new blood vessels and their endothelium by branching preexisting blood vessels. In adults, the proliferation rate of endothelial cells is very low. For example, in mammals, an endothelial cell may divide once per month or may wait several years. It means that angiogenesis is rare in tissues under normal physiology, excepting female reproductive organs. However, it can be activated in pathological processes like tissue damages and tumors. Some substances induce angiogenesis, such as endothelial growth factor (EGF), acidic EGF, fibroblast growth factor (FGF), transforming growth factor (TGF) and prostaglandins. Angiogenesis begins when angiogenic substances are released from surrounding tissues. An increase of VEGF promotes the uptake of glucose through GLUT transporters, which are stimulated and upregulated. Usually, endothelial cells show a very reduced metabolism, but it is also based on glycolysis. Glycolysis makes sense as an energy source because new blood vessels may invade low oxygen regions. The nearest blood vessels that perceive the angiogenic signal relax the attachment between endothelial cells, pericytes lose their contacts and the basal membrane is degraded. The endothelial cell of near blood vessels, usually at a venule, which is exposed to the highest angiogenic signal concentration, starts to migrate to the angiogenic signal source, dragging the other endothelial cells, which begins to proliferate and form a new vessel. This process takes place in several blood vessels at the same time. The elongation and branching of the new blood vessels continue until the vasculogenesis signal disappears. The tips of the blood vessels fuse with other blood vessels to form closed circuits and allowing the flux of blood without dead endings. Macrophages may participate in the fusion process by releasing proangiogenic factors.
Places with endothelial cell progenitors have been found in the dorsal aorta and in the endocardium. Mesenchymal cells can be differentiated from endocardial endothelial cells during the heart development. These cells are able to form the tricuspid valve and some fibroblasts of the heart, but not cardiomiocytes. Endothelial cells may respond to so many external signals that maintaining the endothelium integrity is an active process, which is mediated by signals like FGF. The active process is not just for integrity, but also to keep the cellular phenotype. When endothelial cells do not receive proper signals, they die by apoptosis or become mesenchymal cells that may synthesize abundant extracellular matrix. The transition from endothelial to mesenchymal cell is involved in some diseases like arteriosclerosis and fibrous myocarditis.
3. Function
The idea that the endothelium is just a passive layer of lymphatic and blood vessels has to be changed. Endothelium functions are varied and essential for the organism. Indeed, besides being a physical structure of cardiovascular and lymphatic ducts, it also regulates the exchange of molecules between the lumen of the vessels and the surrounding tissues, and it influences the physiological properties of the blood. Endothelial cells also perform secretory, metabolic and immune functions. They change their physiological behavior influenced by molecules like growth factors, coagulants and anticoagulants, low density lipoproteins, nitric oxide, serotonin, enkephalin, and many others. Endothelial cells bear receptors for all these substances.
Barrier
Endothelial cells form a layer that usually acts as a barrier between the blood and the surrounding tissues. The cohesion between endothelial cells is carried out by cell junctions, such as tight junctions and adherent junctions, but not desmososmes. Gap junctions have also been observed, although their main function is the communication between adjoining cells. Endothelial cells can modulate these adhesion and change the permeability of the endothelium, that may affect not only to molecules but also to cells crossing the endothelium. Perhaps one of the best sealed endothelial barrier is found in the capillaries of the nervous system, as part of the blood-brain barrier. However, in some organs, such as the liver, endothelial cells are loosely packaged and leave much free space, so that endothelium can hardly been regarded as a barrier.
Transport across the endothelium may be classified in four types: passive diffusion (oxygen and liposoluble substances), mediated by transporters (glucose, fatty acids, amino acids), mediated by ABC transporters (uptake metabolites from the nervous tissue and from the blood), and by vesicles. Transmission electron microscopy images show many vesicles in the cytoplasm of endothelial cells. They are thought to be involved in intracellular transport between the apical (facing the blood) and basolateral membranes (facing the basal lamina). This type of transport is known as transcytosis. It is interesting that transcytosis vesicles are more abundant in the endothelial cells of capillaries than in the those of larger blood vessels. It indicates that the endothelial cells in capillaries have a more intense exchange of molecules between blood and tissues, whereas in large diameter vessels they are mainly involved in conducting the blood.
Some endothelial cells, known as fenestrated, have pores or very small ducts that directly connect the blood with the surrounding tissues, allowing small size molecules to cross the endothelium without entering the cytoplasm of any endothelial cells (Figure 4). Fenestrated capillaries can be found in the liver, kidney glomeruli and endocrine structures. Finally, there are organs with very permeable endothelium. In the liver, sinusoids are blood vessels where endothelial cells leave free space between each other, and a function as a barrier hardly exists.
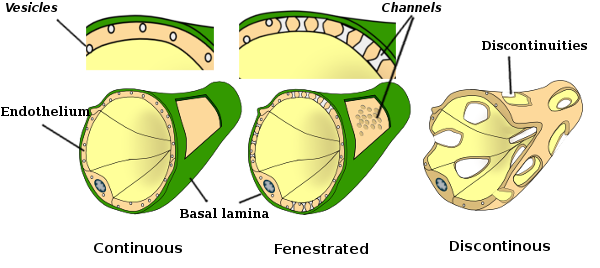
Many cell types travel in the blood toward their target organ in the body. There, they cross the endothelium of the blood vessels, commonly at the level of post-capillary veins. It means that endothelial cells have to modify their cell junctions to let cells go through the endothelium layer. Leukocytes exit the blood vessel by recognizing and anchoring to specific molecules of the apical plasma membrane of the endothelial cells. Adhesion molecules, such as selectins, integrins and immunoglobulins, are responsible for recognition and adhesion of leukocytes to the endothelial layer. Selectins begin the anchoring of leukocytes, which roll over the endothelial surface. This initial adhesion is weak and reversible. If the leukocyte can establish a strong adhesion, the cell is able to cross the endothelium.
Leukocytes exit the blood during inflammatory processes to move to the affected tissues. Chemokines are leukocyte-attractive molecules that are released by damaged tissues and get linked to the glycocalyx of endothelial cells. Leukocytes rolling over the endothelial surface with chemokines are activated, cell-cell adhesions get stronger, and leukocyte remains in the place. The activation of the leukocyte leads to the activation of its integrins, which recognize the immunoglobulins of the endothelial cell. These immunoglobulins are expressed in the endothelial cell membranes after the activation of the cell by chemokines. The cell-cell adhesion raises the calcium concentration within the endothelial cells and leads to the disorganization of the cell junctions between endothelial cells and cytoplasm retraction. In this way, leukocytes can move to the border of endothelial cells and cross the endothelium. Other adhesion molecules are also involved in this movement of leukocytes.
Blood properties
The endothelial functions are more complex than just control the molecules and cells going across the endothelium. For example, endothelial cells are also involved in regulating the blood pressure and clotting.
The primitive circulatory system is thought to have emerged 600 million years ago in invertebrates, but it lacked endothelium. Endothelial cells appeared 100 million years ago, providing blood with a more laminar flux (not turbulent) and therefore a more efficient gas exchange.
Endothelial cells modulate the blood pressure by releasing substances that act on smooth muscle of blood vessels. They release nitric oxide (NO) and prostacyclin, which relax vascular smooth muscle. They also release endothelin and the platelet activator factor, both decreasing the blood vessel diameter. Nitric oxide is constitutively released and provides a proper muscle tone, inhibit platelet aggregation and leukocyte adhesion. Endothelin is a strong vasoconstrictor. How endothelial cells decide what molecule has to be released is not clear yet, but it can be mediated by mechanoreceptors in their apical membranes that are able to feel the flux properties of the blood.
Under normal conditions, endothelial cells release molecules into the blood that help to maintain a proper fluidity. They work at two levels: fluidity (anticoagulants) and preventing platelet aggregation (anti-thrombotic substances). Proteins C and S are important molecules affecting blood fluidity. C protein, forming a complex with S protein, inactivates coagulation factors VIIIa and Va. S protein is synthesized by endothelial cells. Furthermore, the endothelial glycocalyx contains a glycosaminoglycan similar to heparin that is able to inactivate thrombin.
Related to their anticoagulant function, endothelial cells release nitric oxide and prostacyclin, both rise the cyclic AMP in platelets and make more difficult platelet aggregation. These two molecules are continuously released into the blood. Endothelial cells have ectonucleases in their apical membranes, which remove ATP and ADP, both strong promoters of platelet aggregation. In addition, endothelial cells release an activator molecule that transform plaminogen into plasmin, which favor the removing of thrombi.
All these molecular pathways may change when endothelial cells receive some signals or the tissue is damaged, which lead to blood clotting and platelet aggregation. In these circumstances, endothelial cells become active participants of clotting and thrombosis.
Immune defense
Endothelial cells play a major role in the cellular immune defense through two mechanisms: antigen presentation to T lymphocytes and recruiting immune cells. Together with macrophages, endothelial cells can present antigens to T lymphocytes because they constitutively express MHC-I (major histocompatibility complex) and may be induced to express MHC-II, both necessary for antigen presentation. Endothelial cells are able to activate immune memory, but not induce new T lymphocytes. There is bidirectional activation between endothelial cell and T lymphocytes, so that endothelial cell release molecules for attracting inflammatory cells and express adhesion molecules for anchoring blood leukocytes.
-
Bibliografía ↷
-
Bibliografía
Cines BD, Pollak ES, Buck CA, Loscalzo J, Zimmerman GA, McEver RP, Pober JS, Wick TM, Konkle BA, Schwartz BS, Barnathan ES, McCrae KR, Hug BA, Schmidt A-M, Stern DM 1998. Endothelial cells in physiology and in the pathophysiology of vascular disorders. The journal of the american society of hematology. 91:3527-3561
Dejana E, Hirschi KK, Simons M. 2017. The molecular basis of endothelial cell plasticity. Nature communications. 8:14361
Fajardo LF. 1988. The complexity of endothelial cells. American journal of clinical pathology. 92:241-250.
Michiels C. 2003. Endothelial cell functions. Journal of cellular physiology. 196:430-443
-