Intermediate filaments are components of the cytoskeleton. Their main function is to withstand mechanical stress, mainly stretching. This role is clear in animal cells. In plant cells, however, counteracting mechanical forces are carried out by the cell wall. Intermediate filament proteins have been found in plant cells, but their function is not fully understood. It is thought that intermediate filaments appeared in LECA (last eukaryote common ancestor), whereas other cytoskeletal proteins, such as tubulins and actin, arose in LUCA (last universal common ancestor), that is, the last common ancestor of both prokaryotes and eukaryotes.
The diameter of intermediate filaments is 8 to 15 nm, between actin filaments (7 to 8 nm) and microtubules (25 nm). That is why they were named intermediate. Initially, intermediate filaments were thought asto be leftovers of actin filaments and microtubules, so they were the last element to be considered a cytoskeletal component. Intermediate filaments are divided into two systems: cytosolic and nuclear. The cytosolic intermediate filaments are thougth to evolve from the nuclear intermediate filament genes by duplication and mutation. Anywaw, they altogether form a net that spreads from the nuclear envelope to the cell periphery (Figure 1). Cytoplasmic intermediate filaments are usually anchored to adhesion cell complexes such as desmosomes and focal adhesions in cell-cell adhesions and hemidesmosomes in cell-extracellular matrix adhesions. Within the nucleus, they form the nuclear lamina, a scaffold of intermediate filaments that provides shape and consistence to the nuclear envelope and, therefore, to the nucleus as a hole. Intermediate filaments are abundant in those cells under heavy mechanical stress, such as neuronal axons, muscle cells, and epithelial cells.
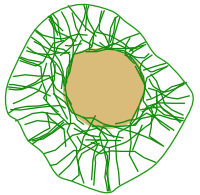
1. Molecular structure
In humans, there are 70 genes coding for proteins that, after polymerization, become intermediate filaments. In addition, mRNA alternative splicing occurs in these genes, giving to a broader protein variety. These proteins contain a globular domain in the C-terminus end, another one in the N-terminus end, and a large central domain in between (Figure 2), altogether containing about 310-350 amino acids that span about 45 nm in length. Globular domains are responsible for interacting with other cellular components and are the most variable part of the intermediate filament proteins. Globular domains also play a role in the spontaneous association between intermediate filament proteins. Thus, polymerization does not need ATP or GTP. The central domain shows an α-helix structure, which allows the association of intermediate filament proteins to form dimers. Two dimers are associated laterally and antiparallel by electrical forces to form tetramers, and tetramers join laterally to form a sheet of 8 tetramers. This sheet rolls over itself to form a coiled structure of about 8 to 10 nm in diameter. In tranverse view, an intermediate filament shows 32 molecules. The 8 rolled tetrameres form the assembling basic unit, which is about 60 nm in length. These basic units line up to form long filaments. The different types of intermediate filaments show proteins with similar central domains, both in size and amino acid sequence, so the diameter and morphology of these filaments are similar.

Intermediate filaments are more stable over longer periods of tims than microtubules and actin filaments. They are also more resistant to high ionic concentrations. However, intermediate filaments can also depolymerize and polymerize by phosphorylation and dephosphorylation, by kinases and phosphatases, respectively, as well as by the activity of some chaperones. There are a few proteins associated with intermediate filaments that influence their activity. Intermediate filaments can be renewed by removing and adding new protein units. Unlike the other two cytoskeletal members, intermediate filaments cannot be used as transport rails for molecules and organelles because they are not polarized, and there are no associated motor proteins. Actually, intermediate filaments are transported along microtubules and actin filaments.
2. Function
The functions of intermediate filaments depend on their molecular composition. They are flexible and resistant, two desirable features for withstanding mechanical stress. They are responsible for the cell integrity of animal cells, and they extend from the plasma membrate to the nucleus. It is estimated that they can be stretched about 250 to 350 % of the resting length because protein units may slide over each other, leading to a smaller diameter of the filament. When the mechanical forces are weak, they work as elastic strands, but they reach a point of no-stretching and become resistant rows under heavy loads. Since intermediate filaments are organized as bundles and nets, they behave as scaffolds with viscoelastic properties. On the contrary, microtubules and actin filaments are quite stiff. The molecular composition of the intermediate filaments determines their mechanical properties and how they associate with one another.
Besides mechanical functions, intermediate filaments are thought to be involved in an array of cellular processes. They may work as anchoring structures for signaling molecules. Intermediate filaments form a scaffold for cellular structures and directly interact with mitochondria, Golgi apparatus and lysosomes, probably affecting their functioning and the vesicular trafficking. For instance, vimentin has been found to interact with Rab proteins, which are necessary for the delivery of vesicles and for placing lysosomes in suitable places. Desmin, queratins and neurofilaments modulate the location of mitochondria. Do not forget that intermediate filaments cannot move cargoes, but they can settle them to a specific place.
The position of the nucleus in the cell depends on the intermediate filaments. The laminas, proteins of the intermediate filaments family that form the nuclear lamina of the nuclear envelope, are connected to the cytoplasmic intermediate filaments through intermediary proteins. Vimentin, desmin, queratin and glial fibrilar acid protein are involved in positioning the nucleus in the cell. For instance, the nuclei of the skeletal muscle cells are found near the plasma membrane thanks to the attachment to desmine intermediate filaments. It is noteworthy that laminas are anchoring points for chromatin, and it has been suggested that mechanical stress on the cell can be translated to chromatin and modulate gene expression.
The intermediate filament scaffold has to be rearranged in some circumstances, such as during cell movement, cell division, or when mechanical forces change the direction. After tissue injury, the expression pattern of intermediate filament proteins also changes near the wound. The net of intermediate filaments is disorganized for apoptosis to progress. Other events affecting the intermediate filament organization are heat stress, osmotic changes, hypoxia, and pathogen invasion.
3. Types
Intermediate filaments can be divided into 6 groups or classes.
Types I and II are acid and basic keratins, respectively. Both types combine between each other to form cellular keratins, meaning that keratins are actually heterepolymeres. Keratins form a family of intermediate filaments having the most variable set of monomers. In humans, there are 54 genes synthesizing different keratin monomers, 28 belongs to type I and 26 to type II.
Keratins are abundant in epithelial cells. There are 17 different keratins in the hair, and the rest of the keratins are epithelial. Different sets of keratin are expressed depending on the epithelial type. For example, stratified squamous epithelium (excluding epidermis and cornea) does not express high molecular-weigh keratins, which are present in the corneum stratum of the stratified squamous keratinized epithelium. There are also particular keratins in the hair, feathers and nails. Whatever the keratin type, it is formed by a mix of different keratin monomers. Keratins are also found in hepatocytes, pancreatic acini, and myoepithelial cells.
Type III is composed of a heterogeneous population of intermediate filaments divided in four groups: vimentins, desmins, glial fibrilar acid proteins (GFAPs), and periferins. Vimentins are expressed in many cell types, such as messenchymal cells, leukocytes, endothelium and some other epithelial cells, usually together with other intermediate filaments. Vimentins are distributed through the cytoplasm and strongly interact with the nucleus. Desmins are major components of the cytoskeleton of the striated skeletal muscle cells. They are not found in myoblasts because they start to be expressed during the fusion of myoblasts to get differentiated muscle cells. Desmins are associated with Z discs of sarcomeres. Glial fibrilar acid proteins are expressed in astrocytes and other glial cells and are made up of just one type of protein. Peripherins are found in cranial nerves and peripheral neurons.
Type IV includes neurofilaments, found typically in neurons, syneimin, syncoilin and alpha-internexin. Regarding their molecular weight, they are classified as light, medium and heavy. Neurofilaments are expressed in mature neurons, are involved in the organization of dendrites and axons, and interact laterally with microtubules and actin filaments. They are made up of three types of monomeres.
Type V intermediate filaments are the nuclear lamins, and they are the only type not found in the cytoplasm. In vertebrates, four genes for type V intermediate filaments have been found: laminas A, B1, B2 and C.
Type VI encompasses a new class of intermediate filaments containing eye lens proteins such as filensin and phakinin. Nestins expressed in the proliferating nerve and muscle cells during development are also included.
4. Pathology
There are more than 80 human diseases associated with intermediate filament defects, such as myopathies, amyotrophic lateral sclerosis, and Parkinson disease. For instance, keratin filaments in the epithelial cells are usually anchored to desmosomes and hemidesmosomes. Epidermoslysis bullosa simplex disease develops after mutations that modify the formation of keratin filaments. It results in a very vulnerable skin to mechanical stress; that is, weak touches may separate the epidermal cells, which are detached very easily.