The extracellular matrix of animal tissues is a molecular network mainly composed of proteins, proteoglycans, glycosaminoglycans, and glycoproteins, that combine to form a variety of extracellular matrices. The more abundant structural proteins are collagen and elastin.
1. Collagen
Collagens form a family of proteins found in animals, so abundant that they can be up to 25 to 35 % of the total protein content of the body. Actually, they are the most abundant proteins in the body. Traditionally, collagen has been used to produce glue and adhesives, and that is why the name collagen (from Greek kolla: glue). In vertebrates, there are about 46 genes that code for polypeptides known as alpha chains. Three alpha chains join to form a triple helix, which is the repeated unit that makes the macromolecular collagen structures. The combination of different alpha chains makes up to 28 types of collagen. Collagen units may be homotypic when the tree alpha chains are the same type, and heterotypic if the triple helix is made up of different alpha chain types. In tissues, the main function of collagen is providing mechanical support and withstand tensile forces, working as the steel bars inside the pillars of buildings. Collagen molecules may be organized in several ways, such as fibers and sheets. Cells bind collagen molecules through a number of plasma membrane adhesion proteins, such as integrins, immunoglobulins, annexins, and some others. Other molecules of the extracellular matrix, like proteoglycans and glycoproteins, are also able to recognize and bind collagen molecules.
Organization
Some features of collagen molecules are the following:
a) A distinct amino acid sequence. Glycine is very abundant in the collagen molecule, together with less abundant hydroxylysine and hydroxyproline. Glycine is found every three amino acids in the sequence (x-y-Gly-x-y-Gly-x-y-Gly-), where Gly is glycine, and x and y are often hydroxylysine and hydroxyproline, respectively. Thanks to the low molecular size of the glycine amino acid, this repeated sequence enables polypeptides to adopt left-handed helix conformations, i.e., alpha chains.
b) Collagen can be arranged in fibers, sheets, or it may work as a linking/anchoring molecule. It depends on the amino acid composition of the alpha chains and on the type of alpha chains in the triple helix.
Fibers. Collagen is mostly arranged in fibers made up of aligned and repeated collagen molecules (Figures 1, 2 y 3). Collagen type I is the most abundant fibrillar collagens. About 90 % of the total collagen of the body. It is abundant in bones, cartilage and skin. Other types of fibrillar collagens are type II, mainly located in the hyaline cartilage, and type III, found in skin and blood vessels. In the same way that there are heterotypic collagen molecules (see above), there are heterotypic collagen fibers, that is, fibers composed of more than one type of collagen. For example, there are collagen fibers consisting of type III and V collagens.


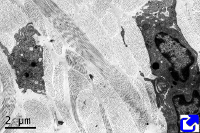
Sheets. There are collagen molecules forming molecular networks arranged in sheets. They are found surrounding organs, in the basal part of epithelial tissues, and around muscle cells. For example, type IV collagen is a main component of the basal lamina located between epithelia and connective tissue.
Beaded filaments. Some collagen molecules are organized as thin filaments with small thickenings that form a net connecting different extracellular molecules. They cross-link molecules and contribute to the extracellular matrix strength. Type VI collagen is an example of collagen forming beaded filaments.
Associated to other collagens. These collagen types interact with other collagen types and help to get a proper orientation, organization and help to interact with other molecules of the extracellular matrix. For example, type IX collagen links glycosaminoglycans and type II collagen fibers.
Moreover, there are some collagen molecules containing sequences of hydrophobic amino acids inserted in the plasma membrane. Type XIII and XVII collagens are transmembrane proteins that function as receptors and are related to cell adhesion and movement. Collagen type XVII is part of the molecular structure of hemidesmosomes.
Synthesis
No matter the type, collagen is synthesized inside the cell as a precursor: the pro-collagen molecule (Figure 4). First, alpha chains are synthesized in the endoplasmic reticulum, where proline and lysine become hydroxylated. Hydroxylysine and hydroxyproline may be up to 20 % of a alpha chain. Glycosylation (type O-glycosylation) of alpha chains also occurs in the endoplasmic reticulum. At this point, three alpha chains join by hydrogen bonds to finally form the pro-collagen molecule. Disulfide bridges are also formed between the alpha chains. Pro-collagen molecules are recognized and linked by transmembrane receptors and packaged in COPII coated vesicles. Typical COPII vesicles are 60 to 90 nm in diameter, but COPII vesicles transporting pro-collagen are 500 nm in diameter because pro-collagen molecules are around 300 nm in length. It is not known how pro-collagen molecules arrive at the Golgi apparatus, cross it, and are released from the TGN to the extracellular space by exocytosis. It is of notice that some cells are able to select different cell membrane domains for releasing different types of collagen. During or after the releasing process, terminal amino acid sequences are enzymatically removed from each alpha chain of the pro-collagen molecules so that pro-collagen becomes collagen molecules. These terminal sequences were preventing spontaneous assembling of collagen molecules into 3D structures within the cell. It was thought that the collagen fibers assembled outside the cell, but it looks like it starts during the exocytosis process.

In fibrillar collagens, free collagen molecules are spontaneously assembled into microfibrills that in turn join to form collagen fibers (Figures 4 and 5). Types V and XI collagens have been suggested to collaborate during the formation of these collagen fibers. Collagen type V appears to be necessary because the fibers are not formed when collagen V is absent. Proteoglycans, such as decorin, fibromodulin and limican, also influence the size and organization of these fibers. During the final assembling stage, fibers get stabilized by enzymes like lysyl oxidase.

Collagen is mainly synthesized by fibroblasts, myofibroblasts, osteoblasts and chondrocytes. However, many other cell types may contribute to extracellular matrix collagen repertory. For example, some collagen molecules are synthesized by epithelial cells.
2. Elastic fibers
Elastin is an abundant and insoluble polymer made up of proteins that form part of the elastic fibers (Figures 6 and 7). The major component of elastin is the protein tropoelastin. Tropoeslastin proteins are released into the extracellular matrix and chemical bonds are formed between one another, forming large aggregates of proteins. Tropoelastin is a large chain of amino acids containing several sequences of hydrophobic amino acids, separated by other sequences containing pairs of glycines and other small amino acids like lysine. This amino acid composition confers the elastic properties: hydrophobic amino acids tend to produce globular structures, and lysine allows alpha-helix structures. Therefore, in aqueous environments, tropoelastin becomes globular, but it can be stretched by mechanical forces. When forces stop pulling, tropoelastin recovers the globular conformation, shrinking the elastic fiber and therefore the tissue. Non-hydrophobic amino acids are the binding points for cross-linking tropoelastin molecules, thus making elastin polymers. The high content of hydrophobic amino acids makes tropoelastin one of the most tough and durable protein of the extracellular matrix. Tropolastin seems to have been invented by vertebrates, since it has not been found in invertebrates.

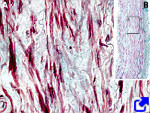
Unlike collagen fibers, elastic fibers can be stretched by mechanical forces and can recover the initial length by shrinking, thanks to the molecular properties of tropoelastin. Elastic fibers are made up of elastin (polymer of tropoelastin), which is up to 90 % of the eleastic fiber, by a coat of fibrillin proteins arranged in fibrills, and other less abundant glycoproteins (Figure 8). The elasticity of our tissues depends greatly on the elastic fibers, which are abundant in the dermis, walls of arteries, and connective tissue of lungs. The amount of elastic fibers is different between tissues. For example, it may be up to 70 % of the extracellular matrix in ligaments. During aging, the proportion of elastic fibers decreases in tissues and organs, and therefore they become stiffer. This is not good for structures like arteries. Besides elasticity, elastic fibers provide support and may regulate cell communication. For example, fibrillin regulates the activity of the transforming growth factor beta (TGF-β).

-
Bibliografía ↷
-
Bibliografía
Heino J. 2007. The collagen family members as cell adhesion proteins. Bioessays. 29:1001-1010.
Canty EG, Kadler KE. 2005. Procollagen trafficking, processing and fibrillogenesis. Journal of cell sciences. 118:1341-1353.
Kadler KE, Baldock C, Bella J, Boot-Handford RP. 2007. Collagens at a glance. Journal of cell science. 120:1955-1958.
Kielty CM. 2006. Elastic fibres in health and disease. Expert reviews in molecular medicine. 8:1-23.
-