The protection tissues constitute the outermost layer of the plant organs, so they are directly exposed to the environment. The epidermis and periderm are the typical protection tissues. The epidermis is present in those organs with primary growth, and the periderm in those with secondary growth. The hypodermis, a tissue layer found just below the epidermis of aerial organs in some plants, is included as protecting tissue, as is the endodermis, which protects the vascular system in the roots.
1. Epidermis

The epidermis is the covering layer of the plant organs (Figure 1). It is accepted that there is no epidermis in the root calyptra and that it is not differentiated in the apical meristems. In addition, the epidermis disappears from those organs undergoing secondary growth, where it is replaced by the periderm (see below). In those plants with only primary growth, the peridermis is present for the lifespan, except in some monocots that replace the epidermis for a kind of periderm. In the stem, the epidermis is developed from the outermost layer of the shoot apical meristem, known as the protoderm. In the roots, the epidermis is differentiated from either the calyptra cells (dicots) or from the outer layer of parenchyma (monocots). This disparity of origins for the epidermis leads some authors to rename the root epidermis as ryzhodermis. During the embryonary development of seeds, a protoderm is formed, even before the shoot and root apical meristems. Then a protodermis is differentiated from the protoderm, which later leads to the epidermis.
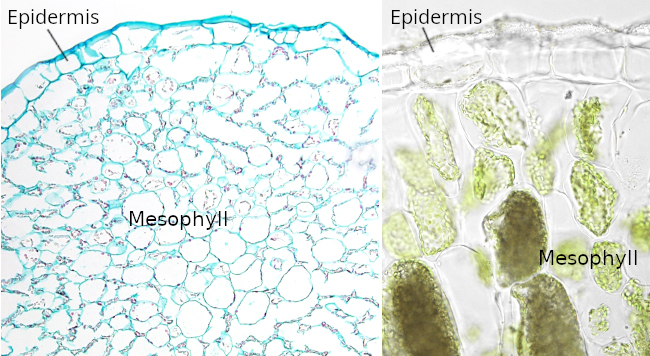
The epidermis is the protective tissue of stems, leaves, roots, flowers, fruits, and seeds. This protection is double, against pathogens and mechanical insults. Other vital functions of the epidermis for plant life are transpiration, gas exchange, storage, secretion, repelling herbivorous, attracting pollinator insects, water absorption in the roots, keeping the physical integrity of some organs, protecting against the sun radiation, and so on. Another rather unknown function of the epidermis is their role in the growth of roots and shoots. The ethylene, in cooperation with auxin, inhibits the growth of organs by preventing the cell elongation. For instance, some environmental stimuli in the roots make ethylene to decrease the elongation of the epidermal cells, and this information is transmitted to inner tissues, decreasing the growth of the whole organ.
Cuticle
The cuticle is a layer found on the free surface, in contact with the environment, of the epidermal cells (Figure 2). It is composed of hydrophobic substances released by the epidermal cells. The cuticle is protective against pathogens and mechanical stress, regulates the salinity tolerance, and protects against low temperatures. It also protects against ultraviolet radiation but allows crossing the light needed for photosynthesis. The cuticle is present in vascular plants, from mosses to angiosperms. It was formed by the land plants, and it probably happened before stomata. The chemical composition of cuticle differs among plant species, but it commonly consists of two components: cutine and waxes (lipids). The molecular substances that form the cuticle of mosses, ferns, gymnosperms, and angiosperms are similar. The thickness of the cuticle is diverse and influenced by the function and location, as well as the environmental conditions (Figure 3). For instance, in Arabidopsis, the cuticle is 80-100 nm thick, 2-9 µm thick in tomato, and in Hakea suaveolens, a xerophyte, it is 11.5 µm. The fleshy fruits show thick cuticles, although they are not more impermeable than the leave cuticles. It is interesting to indicate that the cuticle thickness is not directly related to their hydrophobicity and therefore to prevent water loss. In fact, it is the molecular composition and how the molecules are connected to one another that influence more the permeability of the cuticle. The waxes, more than cutin, are the major substances that influence impermeability. The cuticle composition has also more impact than thickness in preventing ultraviolet radiation. The cutin is actually the scaffold where waxes are laid on.


The cuticle is divided into two layers: the cuticular layer and the lamelar layer. Cutin is abundant in the cuticular layer, or fibrillar layer. Polysaccharides and a large amount of waxes are present in the lamelar layer. Waxes can be found in the cutin matrix (intracuticular) and in the cuticle outer surface (epicuticular) of the cuticle, sometimes in crystal form. It is this epicuticular layer that gives the serous and bright appearance to the leaves. Other less abundant components of the cuticle are cutan (non-saponifiable polymer linked by ether bridges) and a significant amount of other polysaccharides, including cellulose and pectin.
Cutin is a polyester of long fatty acids (16 to 18 carbons) with hydroxyl groups mixed with dicarboxylic acids, alkanoid acids, phenylpropnoids, and glycerol. They altogether work as a scaffold of the cuticle. These molecules are intermingled with waxes. Cutin is synthesized in the endoplasmic reticulum from fatty acids produced in the plastids of epidermal cells. The cutin synthase enzyme is almost exclusively expressed in the epidermal cells. Waxes are a heterogeneous group of molecules that includes a mix of very long-chain fatty acids and their derivatives: alkanes, aldehydes, ketones, alcohols, and esters, as well as cyclic components, such as flavonoids, triterpenoids, and sterols. The actual composition varies according to plant species, plant organs, and even the developmental stage. Environmental conditions may also affect the cuticle composition. It has been estimated that half of the fatty acids produced by protodermal cells are transferred to the cuticle during the differentiation process. The synthesis of waxes begins on the cytosolic surface of the epidermal cell plastids. After further processing, they are transported to the plasma membrane and released into the cuticle by crossing the plasma membrane through ABC transporters. The molecular mechanisms for transporting the waxes and cutin from the endoplasmic reticulum to the cell membrane and how they cross the cell wall are not well known.
Drought increases the release of cuticular waxes. The proportion of alkanes increases more in response to water scarcity. The thickness of the cuticle is also wider. However, as we mentioned, there is no direct relation between cuticle thickness and resistance to dry environments. It is waxes composition that is more effective for preventing water loss.
The root epidermis lacks cuticle, although a very thin layer resembling a cuticle may appear in some species. Since they grow underground, roots are not under a very extreme dry environment, nor radiation. In addition, roots have to favor the absorption of water and soluble substances from the soil through the epidermis.
Cells
The epidermis is commonly formed of one thick cell layer, except for some species showing multilayered epidermis, such as aerial roots, xerophyte plants, and oleander and ficus leaves. These stratified epidermis are also known as multiseriate, or when they are present in the roots as velamen (Figure 4). In multiseriate epidermis, the upper layers work as a typical epidermis, with thick cell walls and cuticles in their free surfaces, while the inner layers may function as water storage.

Several types of cells are found in the epidermis. The epidermal cells proper, or pavement cells, are the most abundant. There are also the idioblasts, which are specialized cells, such as buliforms, glandular, silicic, cork, secretory, and so on. The cells that form the stomata are also components of the epidermis, as well as those forming trichomes and root hairs. The cork and silicic cells may be together, forming pairs. The silicic cells are responsible for the abrasive feature of some grasses. Some cell types are confined to a particular organ, such as petals having cells with pigment and volatile scents and the stigma papilla with cells related to the reception of pollen grains.
The epidermal cells proper are the more abundant and less specialized cells. They are tightly attached to each other, leaving almost no intercellular spaces, and show a broad variety of sizes and shapes that usually follow the shape of the organ they cover. In those elongated organs, as stems, petioles, large leaf veins, leaves of monocots, and some dicots, the epidermal cells proper are also elongated, with their major axis parallel to the major axis of the organ. In dicots, these epidermal cells commonly show sinuous lateral cell walls, but they are more straight in monocots. Although the epidermal cells proper may contain chloroplasts in some aquatic plants and some ferns, they do not commonly contain chloroplasts but other types of plastids. A large vacuole is present in the cytoplasm, as well as the endoplasmic reticulum and Golgi apparatus. A primary cell wall, with variable thickness, is commonly observed. A secondary cell wall is very rare, although it can be observed in the epidermis of seeds and some leaves. However, the secondary well wall is not lignified, except for some gymnosperm leaves. The tangential and inner cell walls bear primary pit fields and plasmodesmata. Channels, referred to as ectodesmata, are observed in the free cell wall, allowing the communication between the cytoplasm and the cuticle and the release of substances toward the exterior.
Other types of cells can be found among the epidermal cells proper; some of them may be used as taxonomic traits. Thus, some epidermal cells are specialized in storing water, such as the bulliform cells of grass leaves and other monocots. Bulliform cells are larger than other epidermal cells, do not contain chloroplasts, show a large vacuole filled with water, and have a scarce cuticle. It has been suggested these cells participate in the leaf folding and unfolding during the cycles of hydration-dehydration. Bulliform cells are ordered in rows parallel to the vascular bundles or in groups in the folding points.
Stomata

Stomata are found in the epidermis of the aerial parts (Figures 5 and 6), mainly in the leaves. They are also found in the epidermis of some stems, petals, and pistils of flowers. The root epidermis lacks stomata, or they are very rare. During evolution, stomata emerged about 400 million years ago, and it is thought that they were invented once. The first land plants lacked stomata, so they could not abandon the aquatic or wet environments. Stomata are the structures responsible for the exchange of gases between the plant and the environment, mostly water and CO2. They are composed of two guard cells that form a pore, or ostiole, between them when they change their shape. Below the guard cells, there is a chamber or empty space known as a substomatic cavity. In most stomata, guard cells are surrounded by specialized epidermal cells called subsidiary cells. The guard and subsidiary cells show different morphology, size, and thickenings of their cell walls. Guard cells, subsidiary cells, and the stomatal cavity form altogether the stomatal complex. Stomata are about 10 to 80 µm large, and there are 5 to 1000 stomata per mm2.


Two main groups of stomata are found regarding the guard cells: in monocot plants they are dumbbell-shaped, whereas in dicot plants they are kidney-shaped (Figures 5, 6, and 7). The grass stomata are claimed to be the most advanced stomata because they are the fastest when alternating between open and closed. Grasses appeared on earth about 55 to 70 million years ago.

Guard cells contain chloroplasts and irregular thick cell. The heterogeneity of the cell wall allows guard cells that changes in the turgor lead to changes in the morphology, which affects the diameter of the stomatal pore (Figure 8). The exchange of gases is largely regulated in this way. However, organs can also increase or decrease the number of stomata in their epidermis, which influence the total gas exchange. The distribution of stomata in the leaves of dicots are more or less scattered through the epidermis, whereas in monocots they are distributed in rows parallel to the leaf veins. This distribution in rows is also observed in pine leaves (Figure 9). The total leaf surface occupies by stomata may be up to 5 %. The stomatal density may be different in the same plant species under, for instance, higher CO2 concentrations may reduce the stomatal density. In addition, different plant organs, or an organ during development, may show changes in the number of stomata. For instance, the fruit epidermis has nearly no stomata, completely lacks stomata or contain non-functional stomata in their mature state. However, during the fruit development, stomata may be necessary for transpiration. In the mature fruit, transpiration is through the cuticle.


The stomatal pore diameter is regulated by water pressure, which is in turn regulated by CO2 concentration, light intensity, hormones as abscisic acid, auxin, cytokinins and gibberellins, pathogen infections, and, of course, the water availability. The guard cells lack plasmodesmata after differentiation, leading to a semiautonomous behavior of each guard cell. The subsidiary cells are responsible for taking from and giving water to the guard cells. In this way, water has to cross both the cell wall and the cell membranes. The plasma membrane may control the flux of water by modifying the ionic gradients. However, the grass stomata show direct cytoplasm-cytoplasm contacts between both guard cells, allowing a synchronized response to turgor changes during the opening or narrowing of the stomatal pore. In general, the larger stomata are less dense and show a weaker response to environmental changes. On the contrary, the smaller stomata show a faster response and generally show higher densities.
Guard cells are usually found at the same height in the epidermal layer than the epidermal cells proper, but not always. In some species, guard cells are protruding from the epidermal surface (see Figure 8), while in others they are below (sunken stomata). Stomata may also be found grouped in epidermal invaginations, referred to as epidermal crypts. The stomata protruding from the epidermal surface are found in species living in humid environments, while sunken stomata are shown by species in arid environments.
There are different ways of stomata formation. Mesogenic: one epidermal cells divides into two new cells, one guard and one subsidiary cell. It is typically found in gymnosperms. Perigenic: guard cell and subsidiary cell are developed from different cells. It is found in angiosperms and many monocots. Mesoperigenic: one of the subsidiary cells is derived from the same cell that the guard cells.
Trichomes and root hairs

Trichomes are unicellular or multicellular appendages that extend from the epidermis of aerial parts of the plant. The word trichome is derived from the Greek word "trichos," which means hair. Practically, all plants have trichomes. They are differentiated from epidermal cells proper. Trichomes are found in leaves and stems and may be present in petals, fruits, and seeds. They may be from several micrometers to centimeters long, and their morphology is diverse depending on the organ and plant species. Trichomes may be classified as glandular and non-glandular, unicellular and multicellular, and branched and non-branched. Botanists have described more than 300 types of trichomes (Figure 10), and these morphological features are sometimes traits for plant species systematics. The plants showing many trichomes are known as pubescent. Most trichomes are formed of living cells, although dead cells may also be found.

Many different types of trichomes, that can be grouped in regions, may be present in the same plant. For instance, in the basal stem of the tomato plant there are long trichomes, whereas they are much shorter in the upper parts. The trichome density may also be different depending on the organ and region. Thus, the density of trichomes is higher on the abaxial (lower) surface of the leaves than on the adaxial (upper) surface. Differences are also observed when comparing plant species. The development of trichomes is influenced by many signals, such as the environment, hormones, regulatory gene activation, and the action of non-coding RNA.
There are protective and glandular trichomes. Protective trichomes (Figure 11) are more frequent in angiosperms. They can be uni- or multicellular. These trichomes protect against intense light and water loss by generating a limit layer over the epidermal surface that reduces the air fluctuations and therefore the water loss. They are very abundant in xerophytes. Other functions are that protective trichomes may guide pollinator insects, influence photosynthesis, regulate the leaf temperature, increase the light reflectance, protect against herbivores, help in seed dispersion and seed protection, and so on. In some species, such as tobacco, trichomes store toxic heavy metals such as zinc and cadmium. Protective trichomes are highly abundant in young organs, from which they may disappear as the organ matures. In some plans leaving on rocks at high altitude, trichomes may absorb water and nutrients from the air. In other species, trichomes may protect against the hits of the sand grains carried by the strong wind against the plant. In aquatic plants, trichomes may help in the floatability because they show some hydrophobicity. Finally, in Arabidopsis, trichomes show thin cell walls and are sensors able to transmit information from the environment to surrounding epidermal cells.

Trichomes may be commercially valuable. The cotton trichomes are a main material for the fabric industry. The trichomes of wood petals keep the structure of the cotton sprouts, ensuring the production of seeds. The tea plant trichomes contain many nutrients. The resistance of some plants to viruses and other pathogens partially depends on trichomes.
Glandular trichomes (Figure 12) contribute to the external secretion on plants. They are commonly multicellular with a stalk, although unicellular glandular trichomes can be found. The stalk consists of cells that anchor the trichome to the basal cells, which in turn attach the whole structure to the epidermal layer. The distal part of the trichome is composed of secretory cells.

Glandular trichomes contain secretory cells that are able to release substances to the environment, either to the air or to the epidermal surface, with different functions. The secretory cells, or glandular cells, show a primary cell wall, sometimes with a cuticle on the surface in contact with stalk cells, a cytoplasm with abundant organelles, such as mitochondria, endoplasmic reticulum, and Golgi cisterns. There are trichomes formed of a basal cell, a stalk cell, and a few secretory cells. These trichomes usually release non-volatile substances that remain on the epidermal surface. Some glandular trichomes show secretory cells with a cavity between the cell wall and the cuticle, which is an extracellular store of secretory compounds.
A broad variety of molecules are synthesized in different types of glandular trichomes, such as organic acids, salts, mucilage (polysaccharides), terpenes, terpene-mucilage mixes, nectar, digestive enzymes released by carnivorous plant trichomes, and so on. One main function of trichomes is to mediate in the animal-plant interactions. Terpenes released by trichomes may produce a variety of responses by insects, such as attraction, repulsion, alarm, or even regulating the animal development, depending on the insect species. Some of the secreted substances may work as antibiotics. Some trichomes protect against herbivores by releasing alkaloids, repellents, or toxic substances.
Root hairs are specialized structures found in root the epidermis. They are involved in the absorption of water and minerals, which also happens in all epidermal cells. Root hairs increase the absorption surface. Some epidermal cells, known as tricoblasts, grow perpendicularly to the epidermal surface, forming a long, hair-like cell. Many symbiotic microorganisms are associated with root hairs, such as nitrogen-fixing bacteria. Root hairs are found in the maturation zone of the root. The number and distribution are characteristic features of plant species, although it may depend on the soil conditions.
2. Periderm

The periderm is a protective structure that replaces the epidermis in stems and roots with secondary growth. It is rare in fruits and leaves. It is usually formed during the first year of secondary growth. However, in some species, the periderm may appear several years after the secondary growth is started. It initially arises from inner tissues of the stem, leading to the detachment of outer tissues. Besides the protective role, the periderm is also a storage compartment for terpenes, alkaloids, phenols, and other bioactive compounds.
The periderm is formed by the activity of the cork cambium, or phellogen, which is a lateral secondary meristem that can arise several times during the lifespan of the tree or woody plant. In the first year of secondary growth, the cork cambium arises for the first time from dedifferentiation of cortical parenchyma cells or collechyma cells found below the epidermis in stems or from the pericycle in roots. It has been reported that it can occasionally also originate from the epidermis and primary phloem of stems. The cork cambium may form a continuous cylinder or a discontinuous circular structure. The tissues that localize more superficially to the cork cambium will die. The first cork cambium may appear several years after germination. For instance, the apple tree forms periderm when it is about 20 years old. In some species, the first cork cambium is the one that lasts all the lifespan of the plant. Most commonly, after the first cork cambium was formed and has been active, which may last several years, a second cork cambium is generated in inner regions of the stem, usually from parenchyma cells of the secondary phloem.
The bark of the trees includes those tissues more superficial than the vascular cambium, that is, the secondary phloem, cortical parenchyma, and periderm. Therefore, it is the result of the activity of vascular cambium and cork cambium. As mentioned above, several periderms can be present in the same stem. All periderms, except the newer one, form the so-called rythidome. Thus, the bark consists of secondary phloem, periderm, and rythidome (Figure 13). There is no rythidome in those plants that develop only one periderm.
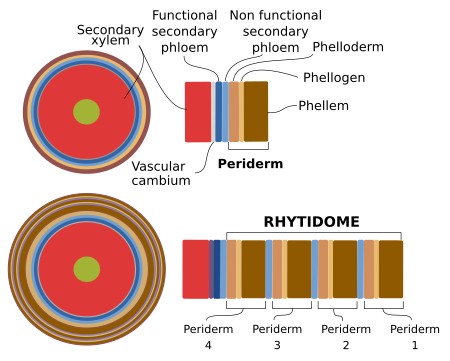
The detachment of tissues outer to the periderm appears to be not just a mechanical process. For instance, the endodermis cells in roots undergo apoptosis, and cortical parenchyma and epidermis degeneration resemble abscission processes. In roots, removing the tissues outer to pericycle during secondary growth is similar to the emergence of lateral roots.
Phellogen cells divide periclinally (see figure), giving rise to layers of cells at both sides, inward the pheloderm, and outward the phellem or cork. The amount of cork is usually larger than that of phelloderm. The cork is made up of stratified cell, morphologically similar to the cell they arise from. They are dead cells that perform mechanical protection, with almost no intercellular spaces, except in lenticels. The cell walls of the cork cells are suberized, and therefore prevent water and gases loss. The genes involved in the suberization of the phellogen derivatives appear to be conserved during evolution. However, some differences may be observed, such as the accumulation of particular metabolites.
There are two types of cells that form cork (phellem) in the periderm: with thick and with thin cell walls. The cells with thick cell walls show a lignified primary cell wall, a suberized secondary cell wall, and an additional inner layer containing waxes, also known as the tertiary cell wall. When waxes fill the inner layer, the cell dies, and the cell performs its mechanical function and prevents water loss. Tannins and resins can be found in these cells, which are imported before they die. The cells with a thin cell wall are larger, with a thinner secondary cell wall, and lack the inner layer. These cells form the cork with commercial value. They may accumulate gas, and they do not contain suberin in their cell walls.
Inward, the phelogen produces living cells, piled in columns, that form the phelloderm. They can be distinguished from the parenchyma cortical cells by their organization in radial stacks. In the first periderm, these cells can perform photosynthesis, and some of them may become differentiated into sclereids.
The periderm is a waterproof layer, mostly the cork layer, that also prevent the gas exchange between the plant and the environment. This barrier may be crossed through the lenticels, structures of the periderm that facilitates the exchange of gases between the inner tissues and the environment. Lenticels are round or lenticular areas with a pore. Beneath the pore, there are parenchyma cells with thin cell walls that leave intercellular spaces. Lenticels are formed concurrently with the first periderm in those regions with a higher phellogen activity. The cells of lenticels are different from the cells of the rest of the periderm. Two types of cells have been described in lenticels: complementary and closing cells. Complementary cells are organized rather loosely, and closing cells are arranged in tangential rows among the complementary cells. The first lenticels are formed under the stomata of the epidermis. As the stem grows, new lenticels are formed. The number and distribution of lenticels depends on the species.
Monocots do not produce periderm or periderm-like layers. The epidermis lasts for the lifespan of the plant, and the underlying layer of parenchyma cells may become suberized or sclerified, forming a protective layer. A "secondary" growth is only observed in a few monocots after the formation of a meristem that differentiates from parenchyma cells. It gives rise to suberized cells that die later. If the stem gets very thick, this process is done again, similarly to what happen to the periderm.
-
Bibliografía ↷
-
Campilho A, Nieminen K, Ragni L. 2020. The development of the periderm: the final frontier between a plant and its environment. Current opinion in plant biology.53: 10-14.
Carptenter KJ. 2005. Stomatal architecture and evolution in basal angiosperms. American journal of botany 92: 1595-1615.
Javelle M, Vernoud V, Rogowsky PM, Ingram GC. 2010. Epidermis: the formation and functions of a fundamental plant tissue. New phytologist 189: 17-39.
Wang X, Shen C, Meng P, Tan G, Lv L. 2021. Analysis and review of trichomes in plants. BMC Plant Biology 21:70. https://doi.org/10.1186/s12870-021-02840-x
Yeats TH, Rose JKC. 2013. The formation and function of plant cuticles. Plant physiology 163: 5-20
Xue D, Zhang X, Lu X, Ghen G, Chen Z-H. 2017. Molecular evolutionary mechanisms of cuticular wax for plant drought tolerance. Frontiers in plant sciences 8: 261
-