1. Morphology
2. Tissues
- Epidermis
- Mesophyll
- Veins
3. Formation
Leaves are generally flat organs derived from shoot apical meristems. They are the major photosynthetic organs, thanks to the high number of chloroplasts that leaf cells contain. In addition, leaves are the main responsible for regulating transpiration, therefore preventing water lost. The design and morphology of leaves can be explained if we take into account these two functions: photosynthesis and transpiration. During evolution, vascular plants probably formed leaves by modifying branches.
1. Morphology
Anatomically, leaves can be divided into two parts: the blade (or limb) and the petiole (Figure 1). The blade is where most photosynthesis and transpiration occur. The greatest part of stomata and photosynthetic parenchyma is found in the blade. There are two surfaces in the blade: the upper, or adaxial surface, and the lower, or abaxial surface. Adaxial surfaces are usually more directly exposed to sunlight, whereas abaxial surfaces are more hidden. During leaf development, the leave primordium has no adaxial-abaxial axis determination, which is established once the leaf emerges from the stem. The leaf polarity can also be observed in the vascular bundles, which become organized with the xylem toward the adaxial surface and the phloem toward the abaxial one. The margin of the leaf, or leaf contour, may show a broad variety of forms. The petiole is more or less long and cylindrical. It connects the blade with the stem at the level of a stem node. Axillary buds, found at the sharp angle between the petiole and the stem, can develop into lateral branches. Some types of leaves, known as sessile leaves, lack petioles and the limb is directly attached to the stem.

The leaf size is variable. Small leaves are often found in higher altitude places, with little rain and poor nutrient soils, as well as in hot and dry places. Solar radiation is another element that affects the leaf size and thickness, even in the same plant body. Leaves under more intense sunlight are smaller and thicker, mostly due to the growing of photosynthetic parenchyma. They also show a more well-developed vascular system and epidermis than shadow leaves.
Regarding the blade complexity, leaves can be divided into simple and compound. Simple leaves have an undivided blade, whereas compound leaves have divided blades into leaflets, sometimes called folioles. Each leaflet looks like a leaf blade, but it is not (Figure 2). Simple leaves and leaflets can be easily distinguished because leaflets have no axillary buds.

The blade shows a large variety of shapes, depending on the species. A particular shape is the result of the adaptation of the species to the environment during evolution. For example, light intensity, rain, temperature, altitude, herbivores, and other factors influence the blade morphology. Different leaf morphologies get different names (Figure 3).

The vascular bundles of the leaf are known as veins or nerves, and venation (or innervation) is how they are organized. Venation can be used to distinguish two main groups of plants. Microphyll plants, such as ferns, show simple venation, whereas megaphylls have more complex vascular bundles organization, like flowering plants. These two types of venation seem to have emerged independently during evolution. It means that they are not homologous. In flowering plants, dicot species usually have a main central vein in the leaf blade that branches many times, and the diameter of the vascular bundle decreases after each branching. This branching pattern is called reticulated (Figure 4). The main vein, known as the rib, extends from the petiole to the tip of the blade through the middle line of the blade. In monoct species, veins run parallel to the long axis of the blade and usually show similar diameters. This organization is called parallel venation. However, there are smaller vascular bundles transversely connecting the parallel larger ones. Although most plants show either reticulated or parellel venation, there are species some species, such as Ginkgo, with a dichotomic venation. The conifers leaves have one or two main veins that run parallel to the major leaf axis. Finally, aquatic plants show a very reduced leaf vascular system.

The general organization of leaf venation depends on the species, but the fine pattern may be influenced by the environment. The position of large veins is more predictable, but the distribution is more diverse as they branch and reduce the diameter, even when comparing leaves from the same plant. In addition, at least in dicot plants, fully developed leaves have some capability to modify the vein organization, for example, to during wound heal.
Petioles of dicot leaves may show only one vascular bundle, which results from the leaf trace of the stem or from the fusion of some of them. Large leaves usually have more than one vascular bundle, one central and several peripheral and smaller. The large vein of the leaves is formed from the large vascular bundle. A cylindrical vascular system may appear in the petiole of the largest leaves. In monocot leaves, petioles show several vascular bundles scattered in the parenchyma.
At the base of the petiole of dicot plants, there is a structure resembling a little leaf or scale called a stipule (Figure 5). In monocot plants, stipule-like structures at the leaf base are wider and usually embrace the stem.

In some species, leaves develop into structures not directly related to photosynthesis. Some leaves are associated with the flowers and form the bracts that are around the petals. Others become thorns, as in hawthorn (but not the bramble thorns which are stem derivatives), or are modified to catch insects as in carnivorous plants.
The presence of leaves in the along the whole main stem and lateral branches happens in plants with a short lifetime, usually one year. However, those species living several years have leaves in the segment of the branch that grew that year, or a few years before. Leaves die and fall in deciduous plants, but remain for several years in perennial ones. New leaves grow on new shoots. The position of leaves in the stem may be spiral when they are arranged as a helix, opposite when two leaves are at the same level and at opposite sites of the stem, or verticillated when three or more leaves are at the same level. Phyllotaxy is the name of the organization of leaves in stems.
2. Tissues
Epidermis

On the adaxial surface (upper surface) of the leaf, there is an epidermis showing a thick cuticle made up of cutin and waxes. Stomata are usually scarce in the adaxial epidermis, or they are not present at all. On the abaxial surface (lower surface), there is a thinner epidermis with a high density of stomata (Figure 6). In xerophyte plants, which are adapted to extremely dry environments, the epidermis cuticle is well-developed and shows abundant cutin. There are leaves, referred to as epistomatic, showing stomata only in the adaxial epidermis, such as floating aquatic plants. Hypostomatic plants are those with stomata only on the abaxial epidermis, and anphystomatic plants show stomata on both surfaces. Finally, the underwater leaves of submerged plants do not usually have stomata. Stomata appear to be randomly distributed through the epidermis of dicot plant leaves. However, in monocot plant leaves, stomata are arranged parallel to the veins. Xerophyte plants usually have a higher density of stomata in the leaves because they allow a large and quick exchange of gases during the short periods of time when water is available.

In the leaf epidermis of many species, there are cells that differentiate into hair-like structures, or trichomes. Trichomes may be unicellular or multicellular, and may have several functions, such as protection, glandular function, and preventing water loss. Leaves lacking trichomes are called glabrous and those with trichomes are known as pubescent. In many monocot species, there are epidermal cells that are involved in the unrolling of leaves when humidity and water are present. They are known as the bulliform cells. In most plant species, epidermal cells lack chloroplasts, except for the stomatal cells. In addition, epidermal cells may have lens-like free surfaces that concentrate the light into the underlying parenchima.
Mesophyll
Mesophyll is the parenchyma found between the adaxial and abaxial epidermis. Most leaves show two types of parenchyma: palisade and spongy. However, it is homogenous in some species. Palisade parenchyma is found close to the adaxial surface, and it is made up of photosynthetic parenchyma cells containing many chloroplasts. They are tidily-arranged, elongated cells, oriented perpendicular to the leaf surface (Figure 6). That is why the name palisade parenchyma. Spongy parenchyma, or aerenchyma, is closer to the abaxial surface, and it is composed of more or less rounded cells with not too many chloroplasts. There are large intercellular spaces in this parenchyma, so it looks like a spongy texture. Intercelullar spaces are connected with substomatic chambers and are important for the gas exchange needed during photosynthesis. However, it is difficult to distinguish between the two parenchyma types in some species. In leguminous and some other dicots, the vascular bundles are connected by layers of parenquimatous tissue to help with the transport of substances between the mesophyll and the vascular bundles.
Dorsiventral leaves are those with a typical distribution of the palisade parenchyma above the spongy parenchyma. Isolateral leaves have two layers of palisade parenchyma (close to the adaxial and abaxial layers, respectively) and a layer of spongy parenchyma in between. Some xerophyte species show isolateral leaves. Homogeneous leaves are those showing an even cell morphology and distribution of parenchyma cells forming the mesophyll, as in grasses and pines.
Many leaves show sclerenchyma and colenchyma beneath the epidermis, organized in a layer known as the hypodermis. This layer contributes to preventing water loss and supporting the leave structure. Sclerenchyma can also be found around the vascular bundles with a mechanical supporting role. Aquatic plants do not have, or show little, sclerenchyma or colenchyma. Secretory ducts can be found in the mesophyll, such as resin ducts in pines, oil cavities in eucalyptus, laticifers, and some others.
Veins
Vascular bundles of leaves are called veins (Figure 7). They bring water and mineral salts to the leaves, and take photosynthesized substances out of the leaves. Xylem and phloem, as well as some parenchyma cells, form the veins. Xylem is usually facing the adaxial surface, whereas phloem is toward the abaxial surface.
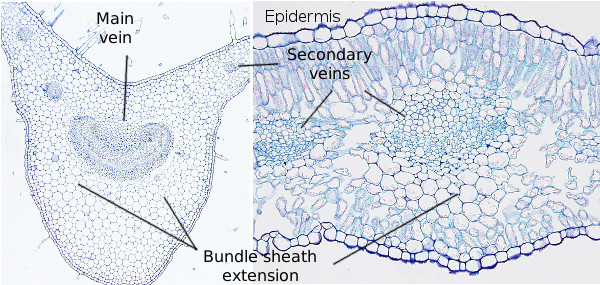
The vascular system of leaves is a network of interconnected veins. Veins show different patterns depending on the species. However, it is a common feature to follow a hierarchical pattern, where veins decrease in diameter after every branching point. Primary and secondary veins are regarded as the main veins because their diameter is higher, and they are wrapped by perivascular parenchyma, or bundle sheath. This wrap may be one or several cells in thickness. Collenchyma and sclerenchyma may also be present. In some angiosperms, the bundle sheath may contain suberized cells, as in the endodermis, which may be a barrier against apoplastic diffusion. Some species show additional parenchymal layers around the bundle sheath, forming what is known as the bundle sheath extension. Lower-order and minor veins are embedded in the mesophyll. There may be sixth-order veins. The lowest-order veins have closed ends. Each type of vein has its own function. Smaller veins are responsible for gathering photosynthesizates from mesophyll, whereas the larger veins are mostly involved in the transport of those substances. Veins also provide mechanical support for the leaf structure. As mentioned before, in some dicot plants with large leaves, veins are surrounded by collenchyma and sclerenchyma cells, whereas monocot may have sclerenchyma fibers associated with veins.
In grasses, there are two types of photosynthetic pathways, C3 and C4. The C4 pathway is more productive. The leaves of both species of grasses show structural differences. C4 leaves show two concentric layers of parenchyma around the vascular bundles. The external one is typical mesophyll, and the internal one is made up of large parenchyma cells containing many chloroplasts (this disposition is known as Kranz anatomy). In addition, the distance between veins is short, no more than the thickness of four photosynthetic parenchyma cells. On the other hand, C3 does not show these concentric layers of cells around vascular bundles, and the bundle sheath is a layer of small cells with few chloroplasts. Inner to the bundle sheath, there is another layer of smaller cells called the mestome. The distance between veins in C3 leaves is larger than 4 cells of photosynthetic parenchyma (around 12, on average).
Endodermis, transfusion tissue

In gymnosperm leaves, there are two additional components that separate the mesophyll from the vascular bundle: the endodermis and the transfusion tissue. The endodermis is a single-cell layer that occasionally shows a Casparian strip and functions much like the root endodermis, that is, regulating the flux of water-dissolved molecules through the apoplastic pathway (although the Casparian strip is impermeable in roots). Furthermore, it has been suggested that the endodermis prevents ice formation and provides flexibility to the whole filiform structure of the leave. The transfusion tissue includes parenchymatic cells and specialized tracheids. These tracheids are dead cells involved, together with the endodermis, in the download of the xylem, whereas the living parenchyma cells establish a bridge between the phloem Strasburger cells and endodermis cells. Pine leaves also have resiniferous ducts.
3. Formation
Leaves are developed from the apical shoot meristems, and from the tip meristems of branches. Leaves differentiate from the lateral cells of these meristems, known as founder cells, which undergo periclinal division and are found in the sub-superficial part of the lateral region of the meristems. The initial number of founder cells may go from 10 to 100. The initial process of leave formation can be divided into three steps: initiation, growing (leave protrusions and leaf primordia are formed), and expansion and maturation (it is mostly an expansion to form the leaf blade). The growth of the leaf is mainly caused by intercalary proliferation of the cells, that is, by division of the cells found between the tip and the base of the leave. The increase in size of the cells also contributes to the final size of the leave. It must be pointed out that a leaf is a complex organ because it has three axes: proximal-distal, adaxial-abaxial, and medial-lateral. Cells must know where they are during the differentiation process.
In the leaf primordium, procambium cells are differentiated from the ground tissue near the epidermis. They are induced by a high concentration of the auxin hormone and are then organized in cords to finally form the veins. Veins are not generated by growing of the cords, but by the addition of new procambium cells. The molecular mechanism is known as auxin canalization. It relies on the fact that differentiated vascular cells conduct auxin better, which induces the differentiation of new vascular cells.
-
Bibliography ↷
-
Bar M, Ori N. 2014. Leaf development and morphogenesis. Development 141, 4219-4230.
Dkhar J, Pareek A. 2014. What determines a leaf's shape? Evodevo 5:47.
Pongrac P, Baltrenaite E, Vavpetič P, Kelemen M, Kladnik A, Budič B, Vogel‑Mikuš K, Regvar M, Baltrenas P, Pelicon P. 2019. Tissue-specific element profiles in Scots pine (Pinus sylvestris L.) needles. Trees. 33:91-101.
-