1. Permeability
2. Fluidity
3. Heterogeneity
- Inner
- Outer
- Bending, thickness
Cell membrane features are determined by their molecular composition. This page deals with three of them: permeability, fluidity and lateral heterogeneity.
1. Permeability
MMembrane permeability is a consequence of the internal hydrophobic environment generated by the lipid fatty acid chains, which is difficult to cross by molecules having a net electric charge. Thus, by preventing free diffusion of some molecule types, membranes can form inner compartments and a distinct internal environment separated from the outer one. Permeability is selective. Polarity and size are the more important molecular features that influence the ability to cross cell membranes (Figure 1). Even water has some restrictions when crossing membranes. The ability of large uncharged molecules, such as glucose, to cross membranes is low. Ions and large proteins with net electric charge can hardly cross membranes.

The unequal distribution of ions and molecules between both sides of a membrane makes electrochemical gradients. The difference between the inner and the outer concentration of electric charges is known as membrane potential. This gradient is used for many cell functions, such as ATP synthesis and transmission of information along the nerves. Semipermeability is also responsible for osmotic processes, which are water movements across membranes from a less concentrated solution in one side to a more concentrated solution in the other side, in order to equal both concentrations. In this way, plant cells are able to increase in size. Molecules that do not cross membranes freely are useful for cells because they can create gradients that may work as informational mechanisms or as energy stores. Cells have transmembrane proteins that are regulated gates to cross the membrane or move charged molecules and ions between both sides of membranes, avoiding the inner membrane hydrophobic environment. For example, muscle contraction is triggered by the opening of channels that break an existing ionic gradient. Membrane permeability depends on lipid composition. More fluid membranes are more permeable.
2. Fluidity
Fluidity is a property of membranes related to the ability of molecules to move inside the membrane. Higher fluidity means that movements are more frequent. There are two type of movements: lateral, i.e., in the same hemilayer, and flip-flop, i.e., jumping from one hemilayer to the other (Figure 2). The lateral movement is much more frequent than flip-flop. Flip-flop movements are really infrequent because the hydrophilic head of the lipid molecule must cross the internal layer of fatty acid chains.

Chemical composition of membranes determines the membrane fluidity. Generally, shorter fatty acid chains and higher amount of unsaturated bonds between carbons of fatty acids increase membrane fluidity. The amount of cholesterol also influences the membrane fluidity. However, the net effect depends on temperature and type of lipids in the membrane. In general, an increase in cholesterol concentration decreases membrane fluidity, although at low temperatures the effect is the opposite. In addition, cholesterol provides membranes with another property known as hydrophobicity, which makes membranes more impermeable.
Cells can modify the membrane fluidity by changing its chemical composition. For example, mitochondria membranes contain cardiolipin, an unsaturated phospholipid that increases hydrophobicity, but does not reduce much the membrane fluidity.
3. Heterogeneity
Because of fluidity, it can be thought that molecules are randomly distributed in membranes and, therefore, they are homogeneous regarding the molecular distribution. This is not true. There are restrictions to the lateral diffusion of molecules that causes membrane heterogeneity, which means that there are regions of a membrane with different molecular composition.
Lateral movement of molecules are restricted by several mechanisms: interactions with the cytoskeleton or extracellular matrix, interactions of membrane molecules between each other, different densities of local membrane areas (changes in fluidity), amount of electrical charges, membrane curvature, and different thickness of membrane domains (Figure 3).

Inner interactions
Interactions of membrane molecules between each other constrain lateral movement (Figure 4). Proteins and lipids lateral distribution are influenced by these interactions.
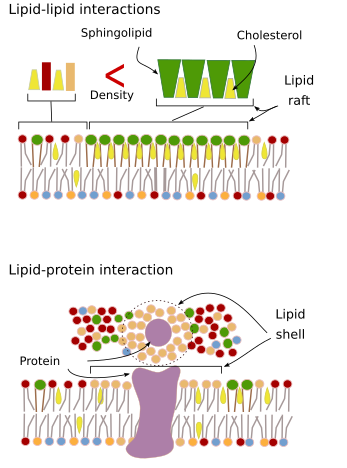
Sphingolipids and cholesterol may become spontaneously associated to one another, reducing their movements and increasing the molecular density when compared to neighbor areas. A small, distinct group of molecules is then formed like a raft in a lower dense sea of lipids. Actually, these molecular associations are known as lipid rafts, and are thought to be very abundant in cell membranes. Lipid rafts show a highly dynamic behavior, they can move, grow, shrink, appear and disappear. Indeed, membranes are made up of many dense domains traveling laterally among the glycerophospholipids, so that they are quite heterogeneous regarding their lateral molecular distribution.
In the inner hemilayer of the plasma membrane, microdomains are formed by electrostatic interactions between the basic cytosolic domains, or divalent cation domains, of proteins and the negative polar heads of lipids. Membrane proteins, both integral and associated, may also interact between each other and assemble into macromolecular scaffolds to facilitate transmission of information, cell-cell recognition, start some enzymatic activities, and cellular movement.
Interactions with outer elements
Integral membrane protein movements are more restricted than lipids, mostly due to interactions with the extracellular matrix and cytoskeleton through their extracellular and intracellular domains, respectively (Figure 5). These interactions may keep proteins in small areas of the membrane during more time than just by diffusion. Cytoskeleton may form fences just below the plasma membrane that keep proteins restricted in small areas. Cells have other mechanisms to confine proteins into specific domains.
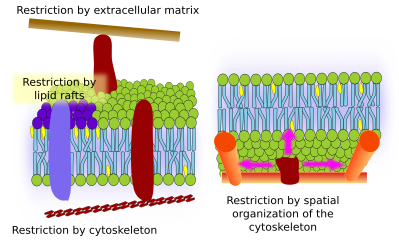
Bending and thicknes
Dending a membrane is another way of generating membrane domains. The curvature may be the beginning of forming a vesicle, generating a cellular extension, changing or growing an organelle, or just a membrane fold. In any case, the curvature works as a barrier to the lateral diffusion of membrane molecules.
Lipid microdomains recruit proteins that effectively bend the membrane. These microdomains are enriched in particular types of lipids, such as phosphoinositides, or show a distinct electric environment that attract bending-membrane proteins (Figure 6).
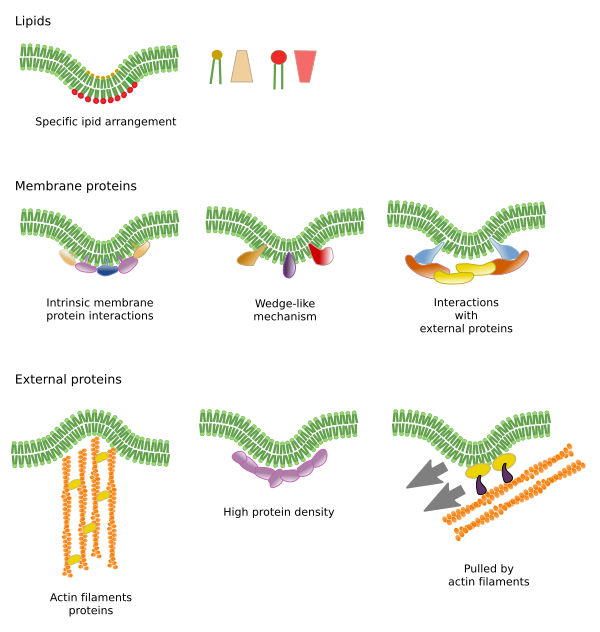
There are other domains in membranes, physical domains, formed by molecules like transmembrane proteins. These areas show different membrane thickness because they contain proteins with longer hydrophobic amino acid sequences that get surrounded by lipids with longer fatty acid chains (Figure 7). Thus, proteins and lipids "feel" more comfortable when they are together since they fit properly their hydrophobic parts.

-
Bibliography ↷
-
Bibliography
Honigmann A, Pralle A. 2016. Compartmentalization of the cell membrane. Journal of mollecular biology. 428: 4739-4748.
Jacobson K, Liu P, Lagerholm BC. 2019. The lateral organization and mobility of plasma membrane components. Cell. 177: 806-819. DOI: 10.1016/j.cell.2019.04.018.
Jarsch IK, Daste F, Gallop JL. 2014. Membrane curvature in cell biology: an integration of molecular mechanisms. Journal of cell biology. 214: 275-387
Nicolson GL. 2014. The Fluid—Mosaic Model of Membrane Structure: Still relevant to understanding the structure, function and dynamics of biological membranes after more than 40 years. Biochimica et biophysica acta. 1838: 1451-1466.
Subczynski WK, Pasenkiewicz-Gierula M, Widomska J, Mainali L, Raguz M. 2017. High cholesterol/low cholesterol: effects in biological membranes: a review Cell biochemstry and biohysic. https://doi.org/10.1007/s12013-017-0792-7
-